While microevolution refers to changes in the allele frequencies of populations, macroevolution focuses on the formation of new species (speciation) and on the evolutionary relationships among groups of species. To understand how the primates evolved, we must first look at how the evolutionary forces discussed in Chapter 2 can lead to macroevolutionary change. As noted in that chapter, the term species is usually defined as a population or group of populations that is capable of interbreeding and producing fertile, viable offspring. In other words, species are reproductively isolated. This definition, however, is not altogether satisfactory, because in nature isolated populations may be in the process of evolving into different species, and it is hard to tell exactly when they become biologically distinct without conducting breeding experiments. Furthermore, this definition can only be tested among living groups. Breeding experiments cannot be conducted with sets of fossilized bones.
Certain factors, known as isolating mechanisms, can separate breeding populations and lead to the appearance of new species. Because isolation prevents gene flow, changes that affect the gene pool of one population cannot be introduced into the gene pool of the other. Random mutation may introduce new alleles in one of the isolated populations but not in the other. Genetic drift and natural selection may affect the two populations in different ways. Over time, as the two populations come to differ from each other, speciation occurs in a branching fashion known as cladogenesis (Figure 6.1) (from the Greek klados, meaning “branch” or “shoot”).
Some isolating mechanisms are geographic—preventing contact, hence gene flow, between members of separated populations. Biologic aspects of organisms can also serve as isolating mechanisms. For example, early miscarriage of the hybrid offspring or sterility of the hybrid offspring, as in the case of closely related species such as horses and donkeys (producing sterile mules), serve as mechanisms to keep populations reproductively isolated from one another.
Isolating mechanisms may also be social rather than physical. Speciation due to this mechanism is particularly common among birds. For example, cuckoos (birds that do not build nests of their own but lay their eggs in other birds’ nests) attract mates by mimicking the song of the bird species in whose nests they place their eggs.
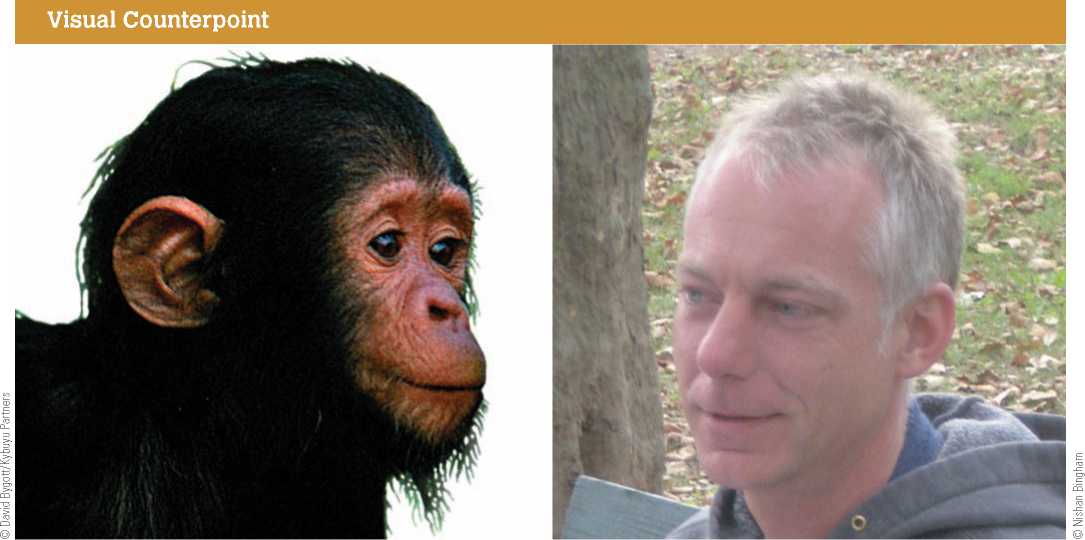
Regulatory genes turn other genes on and off. A mere change in their timing can cause significant evolutionary change because these genes can alter the course of an individual organism's development. This may have played a role in differentiating chimps and humans; for example, adult humans retain the flat facial profile of juvenile chimps. Within primate species in which sexual dimorphism is high, females tend to retain the juvenile traits more than males.
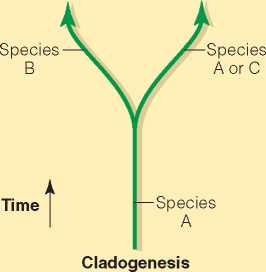
Anagenesis
Figure 6.1 Cladogenesis occurs as different populations of an ancestral species become reproductively isolated. Through drift and differential selection, the number of descendant species increases. By contrast, anagenesis can occur through a process of variational change that takes place as small differences in traits that, by chance, are advantageous in a particular environment accumulate in a species' gene pool. Over time, this may produce sufficient change to transform an old species into a new one. Genetic drift may also account for anagenesis.
Thus cuckoos that are physically capable of mating may be isolated due to differences in courtship song behavior, which effectively isolates them from other cuckoos singing different tunes. Though social rules about marriage might be said to impose reproductive isolation among humans,
—Species B
—Species A
These social barriers have no biological counterpart. For humans, there are no sufficiently absolute or long-lasting barriers to gene flow.
Because speciation is a process, it can occur at various rates. Speciation through the process of adaptive change to the environment as proposed in Darwin’s Origin of Species is generally considered to occur at a slow rate. In this model, speciation may occur as organisms become more adapted to their environmental niche. Sometimes, however, speciation can occur quite rapidly. For example, a genetic mutation, such as one involving a key regulatory gene, can lead to the formation of a new body plan. Such genetic accidents may involve material that is broken off, transposed, or transferred from one chromosome to another.
Genes that regulate the growth and development of an organism can have a major effect on its adult form. Developmental change in the timing of events, a phenomenon known as heterochrony (from Latin for “different time”), is often responsible for changes in the shape or size of a body part. A kind of heterochrony called neotony, in which juvenile traits are retained in the adult state, may be responsible for some of the visible differences between
Heterochrony Change in the timing of developmental events that is often responsible for changes in the shape or size of a body part.
Sometimes mutations in a single gene can cause reorganization of an organism's body plan. Here the “bithorax” homeobox gene has caused this fruit fly to have two thoraxes and two sets of wings. Another homeobox gene, “antennepedia,” caused legs to develop in the place of antennae on the heads of fruit flies.
Humans and chimps. Scientists have discovered certain key genes called homeobox genes that are responsible for large-scale effects on the growth and development of the organism. If a new body plan happens to be adaptive, natural selection will maintain this new form for long periods of time rather than promoting change.
Paleontologists Stephen Jay Gould and Niles Eldred proposed that speciation occurs in a pattern of punctuated equilibria—the alternation between periods of rapid specia-tion and times of stability. Often this conception of evolutionary change is contrasted with speciation through adaptation, sometimes known as Darwinian gradualism. A close look at genetic mechanisms and the fossil record indicates that both models of evolutionary change are important.
Homeobox gene A gene responsible for large-scale effects on growth and development that are frequently responsible for major reorganization of body plans in organisms. punctuated equilibria A model of macroevolutionary change that suggests evolution occurs via long periods of stability or stasis punctuated by periods of rapid change. anagenesis A sustained directional shift in a population’s average characteristics.
All working evolutionary scientists—including Gould, the champion of the punctuated equilibrium model— recognize the importance of both rapid change and gradual Darwinian processes. Gould describes Darwinian evolution as variational change that occurs
By the twofold process of producing copious and undirected variation within a population and then passing along only a biased (selected) portion of this variation to the next generation. In this manner, the variation within a population at any moment can be converted into differences in mean values (average size, average braininess) among successive populations through time.1
He states that this kind of change is unsettling because it is not predictable and does not proceed according to simple natural laws such as gravity. Instead,
The sensible and explainable but quite unpredictable nature of the outcome (dependent upon complex and contingent changes in local environments), the nonprogressive character of the alteration (adaptive only to these unpredictable local circumstances and not inevitably building a “better” organism in any cosmic or general sense)—flow from the variational basis of natural selection.72 73
Genetic mechanisms underlie both rapid and gradual changes because mutations can have small or large effects. It is particularly interesting to see how molecular genetics supports Darwinian evolutionary change. For example, the tailoring of beak size and shape to diet among finches on the Galapagos Islands, in the Pacific Ocean west of Ecuador, constituted Darwin’s classic example of natural selection (Figure 6.2). Recently scientists identified two proteins along with the underlying genes that control beak shape and size in birds. It is all the more impressive that Darwin was able to make his inferences about natural selection without the benefit of molecular genetics.
A fundamental puzzle in the fossil record is that scientists have not been able to pinpoint the precise moment when variational change leads to the formation of a new species. More recent populations may appear sufficiently changed from ancestral populations to be called different species. The difficulty arises because, given a reasonably good fossil record, one species will appear to grade into the other without a clear break. This gradual directional change over time, called anagenesis, can occur within a single line, without any evident branching (see Figure 6.1). Speciation is inferred as organisms take on a different appearance over time.
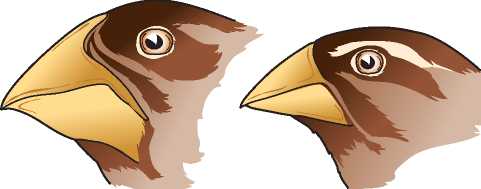
Geospiza magnirostris
Geospiza fortis
Certhidea olivacea
Geospiza parvula
Figure 6.2 Scientists have begun to unravel the genetic mechanisms controlling beak shape and size of finches studied by Darwin on the Galapagos Islands. Darwin noted how beak size and shape were related to each species' diet and used the birds to illustrate adaptation to a particular ecological niche. Finches with blunt crushing beaks are seed eaters while others with long probing beaks pick between cactus thorns for food or use the beaks to get insects.
It may be difficult to determine whether variation preserved in the fossil record presents evidence of separate species. How can we tell whether two sets of fossilized bones represent organisms capable of interbreeding and producing viable fertile offspring? Paleoanthropologists use as many data sources as possible, checking the proposed evolutionary relationships in order to approximate an answer to this question. Today, paleoanthropologists use genetic data as well as observations about the biology and behavior of living groups to support theories about speciation in the past. Thus reconstructing evolutionary relationships draws on much more than bones alone. Fossil finds are always interpreted against the backdrop of scientific discoveries as well as prevailing beliefs and biases. Fortunately, the self-correcting nature of scientific investigation allows evolutionary lines to be redrawn in light of all new discoveries and more compelling explanations.
Constructing Evolutionary Relationships
In addition to designating species in the fossil record, pa-leoanthropologists and paleontologists construct evolutionary relationships among fossil groups. Scientists pay particular attention to features appearing more recently in evolutionary history that are unique to a line, calling these features derived. The counterparts to derived traits are ancestral characteristics, which occur not only in the present-day species but in ancestral forms as well. For example, bilateral symmetry, a body plan in which the right and left sides of the body are mirror images of each other, is an ancestral trait in humans. Because it is a characteristic of all vertebrates including fish, reptiles, birds, and mammals, bilateral symmetry does not contribute to the reconstruction of evolutionary relationships among fossil primates. Instead, paleoanthropologists pay particular attention to recently evolved derived features in order to construct evolutionary relationships among fossil groups. For example, because changes in bones associated with bipedalism are present only in the human line, these derived features can be used to separate humans and their ancestors from other hominoids.
Sorting out evolutionary relationships among fossil species may be complicated by a phenomenon called convergent evolution, in which two more distant forms develop greater similarities. The classic examples of convergence involve analogies discussed in Chapter 2, such as the wings of birds and butterflies, which resemble each other because these structures serve similar functions. Convergent evolution occurs when an environment exerts similar pressures on distantly related organisms causing these species to resemble each other. Distinguishing the physical similarities produced by convergent evolution from those resulting from shared ancestry may be difficult, complicating the reconstruction of the evolutionary history of any given species.
Among more closely related groups, convergence of homologous structures can occur, as when an identical structure present within several distinct species takes on a similar form in distantly related groups. Among the primates, an example is hind-leg dominance in both lemurs and humans. In most primates, the hind limbs are either shorter or of the same length as the forelimbs. Lemurs and humans are not as closely related to each other as are humans and chimps, for example, but both have longer hind limbs related to their patterns of locomotion. Humans are bipedal while lemurs use their long legs to push off and propel them from tree to tree. Hind-leg dominance appeared separately in these two groups and is not indicative of a close evolutionary relationship. Only shared derived features can be used to establish relationships among groups of species.
The Nondirectedness of Macroevolution
In the nonscientific community, evolution is often seen as leading in a predictable and determined way from one-celled organisms, through various multicelled forms, to
Derived Characteristics that define a group of organisms and that did not exist in ancestral populations. ancestral Characteristics that define a group of organisms that are due to shared ancestry.
Convergent evolution In biological evolution, a process by which unrelated populations develop similarities to one another due to similar function rather than shared ancestry.
Visual Counterpoint
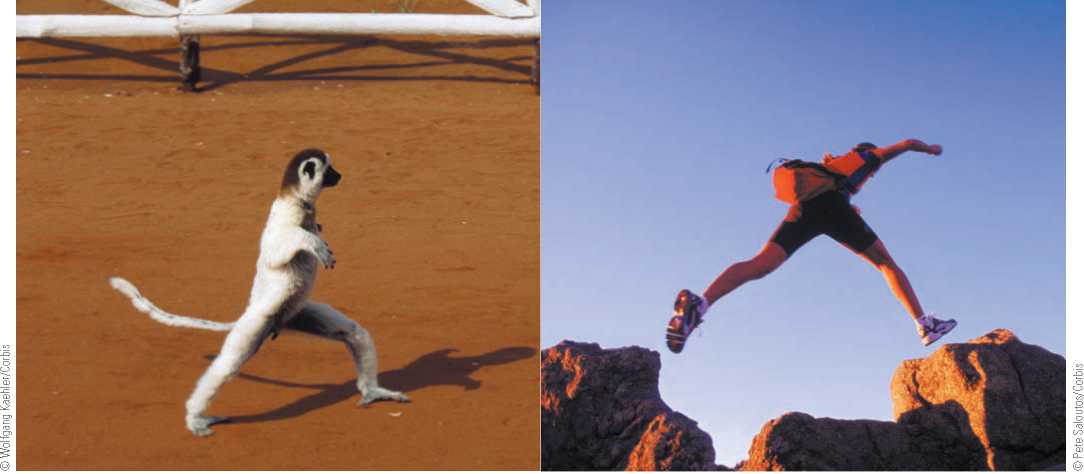
The characteristic long legs of prosimians and humans are not the result of a close evolutionary relationship. This is instead the result of convergence of homologous structures. The long legs of prosimians allow them to follow their characteristic pattern of locomotion, called vertical clinging and leaping. On rare occasions for the briefest periods of time, they are also capable of taking a bipedal step or two.
Humans, who occupy the top rung of a ladder of progress. However, even though one-celled organisms appeared long before multicellular forms, single-celled organisms were not replaced by multicellular descendants. Single-celled organisms exist in greater numbers and diversity than all forms of multicellular life and live in a greater variety of habitats.74
As for humans, we are indeed recent arrivals in the world (though not as recent as some new strains of bacteria). Our appearance—like that of any kind of organism— was made possible only as a consequence of a whole string of accidental happenings in the past. To cite but one example, about 65 million years ago the earth’s climate changed drastically. Evidence suggests that a meteor or some other sort of extraterrestrial body slammed into earth where the Yucatan Peninsula of Mexico now exists, cooling global temperatures to such an extent as to cause the extinction of the dinosaurs (and numerous other species as well). For 100 million years, dinosaurs had dominated most terrestrial environments available for vertebrate animals and would probably have continued to do so were it not for this event. Although mammals appeared at about the same time as reptiles, they existed as small, inconspicuous creatures that an observer who came to earth from outer space would probably have dismissed as insignificant.
But with the demise of the dinosaurs, all sorts of opportunities became available, allowing mammals to begin their great expansion into a variety of species including our own ancestors, the earliest primates. Therefore, an essentially random event—the collision with a comet or asteroid—made our own existence possible. Had it not happened, or had it happened at some other time (before the existence of mammals), we would not be here.75
The history of any species is an outcome of many such occurrences. At any point in the chain of events, had any one element been different, the final result would be markedly different. As Gould puts it, “All evolutionary sequences include. . . a fortuitous series of accidents with respect to future evolutionary success. Human brains and bodies did not evolve along a direct and inevitable ladder, but by a circuitous and tortuous route carved by adaptations evolved for different reasons, and fortunately suited to later needs.”5
Given that humans arrived here by chance relatively late in the history of life on earth, the drastic changes humans have imposed on the environment and on the other species with which we share this earth are particularly shocking. As described in this chapter’s Original Study by Australian zoologist Sir Robert May, it is now time for humans to create solutions.
4 Gould, S. J. (1985). The flamingo’s smile: Reflections in natural history (p. 409). New York: Norton.
5 Ibid., p. 410.

Melding Heart and Head by sir Robert May
Today we are living at a very special time in the history of the Earth. It is a time which might come in the history of any inhabited planet, when the activities of one particular species—in this case, ourselves—come to rival the scale and scope of the natural processes which built, and which maintain, the planet's biosphere.
It is easy to be skeptical of such dramatic claims, which are often voiced around millennia or other years with many zeros in them. But there are objective facts which demonstrate just how special our own time is. For one thing, humans today take for their own use somewhere between a quarter and a half of all plant material that grows on earth each year. For another—from the tropical rainforests, across the grain fields of America, Europe and Asia, to the Arctic tundra—fully half of all the atoms of nitrogen and of phosphorous annually fixed in new plants come from human intervention in the form of fertilizers rather than natural cycles. Turning to the sea, we take 10 percent of all its production each year, and larger amounts (around 30 percent) in rich areas of nutrient upwelling.
But all this represents bad news for the diverse populations of invertebrates, birds, and other creatures that share the countryside with us. The State of the UK's Birds 1999, recently published by the Royal Society for the Protection of Birds (RSPB) and the British Trust for Ornithology (BTO), for example, documents declines in populations of 41 species of woodland birds (on average down 20 percent from the mid-1970s) and of 20 species of farmland birds (down 40 percent over the same period).
More broadly, the outcome of intensification of agriculture, around the world, is an ever more Silent Spring. Documented extinctions of bird and mammal species over the past century or so are at a rate roughly 1,000 times faster than the rates seen, on average, over the half-billion-year sweep of the fossil record. The various causes are habitat destruction, unsustainably excessive harvesting and other exploitation, adverse impacts by introduced alien species, and—more often—combinations of all three.
Projections of future extinction rates are more difficult to make. Four different lines of argument, ranging from one which applies generally to all plants and animals, through to others which generalize from particular families of birds, reptiles, and mammals, all suggest a roughly tenfold increase in extinction rates over the coming few centuries. These are sober, analytic estimates, free of the rhetorical exaggerations which sometimes afflict the subject. These estimates make it clear that we are currently on the breaking tip of a sixth great wave of extinction in the history of life on Earth, fully comparable with the Big Five in the fossil record, such as the one that extinguished the dinosaurs.
Diminishing Gains
Toward the end of this century, estimates which I rate as rather optimistic suggest that—barring catastrophes—our descendants will live in a world of 10 billion people. How will they be fed? The Green Revolution, underpinned as it is by massive and unsustainable inputs of fossil fuel energy, already shows signs of diminishing gains. Just as we could not feed today's global population with yesterday's agriculture, I do not believe we can feed tomorrow's population with today's.
But if we seek only further intensification of agriculture—a further ratcheting up in the spirit of the Green Revolution—then we may feed tomorrow's world, but it will be biologically impoverished, and I doubt its sustainability. If, on the other hand, we use our increasing understanding of the molecular machinery of life, along with other cultural changes, to produce an agriculture that works with the grain of nature—rather than using fossil fuel subsidies to wrench nature to our crops—then I hope we can achieve Conway's Doubly Green Revolution.
Harnessing Impulse
Part of the motive for all this must be a more sustainable way of doing things. But a related part of the motive must come from our natural impulses of concern, and even affection, for the other creatures we share the world with. Too often, however, such concern expresses itself through a disproportionate focus on large mammals and colourful birds: “charismatic megafauna.” Although understandable and effective in engaging a wider public, particularly in the developed world, these targets are not necessarily those that would be chosen in an analytic quest to preserve the maximum amount of the planet's evolutionary history, as written in the genetic richness and variability within today's living species. Although our emotions may relate most easily to the big mammals and the interesting birds, the smaller invertebrates and the diverse plant kingdom are more important for the functioning of many ecosystems, and they also carry more of the record of how life evolved on our planet. The justification that by saving charismatic megafauna we necessarily save large areas of habitat, and thence a host of less emotionally resonant invertebrates and plants, does not always survive close examination: such studies as do exist suggest that “hot spots” for birds are often weakly correlated with “hot spots” for particular plant and insect groups.
To summarize, I believe the challenge of the century is to emphasize valid emotional and ethical arguments for conserving biological diversity, but also to combine them with analytic approaches that ask questions—often cold and difficult ones—about which actions will, in the long run, be most effective in sustaining as much as possible of the biological riches and the unaccounted ecosystem services we have inherited. This melding of heart and head will, I think, pose tough challenges and choices. It is not an easy recipe for a new beginning to a new millennium.
Adapted from May, R. (2000). Melding heart and head. Beyond 2000. New York: United Nations Environment Programme. http://www. unep. org/ourplanet/ Imgversn/111/may. html