Archaeobotany The recovery and identification of plant remains from archaeological contexts, which assist in reconstructing past environments.
C3 plant A plant that produces the 3-carbon compound phosphoglyceric acid as the first stage of photosynthesis.
C4 plant A plant that produces the 4-carbon compound oxalocethanoic (oxaloacetic) acid as the first stage of photosynthesis.
Microbotanical remains Those plant remains from
Archaeological sites that are visible to the naked eye, primarily seeds and charcoal.
Paleoenvironmental reconstruction Investigations which are undertaken to reconstruct the climate and vegetation of a specific time and place.
Phytoliths Minute particles formed of mineral matter by a living plant and fossilized in rock.
Phytolith analysis is one of the most versatile of all the techniques used in archaeobotany. Researchers have used phytoliths to answer both traditional and nontraditional archaeobotanical questions, within archaeological as well as palaeoenvironmental sampling contexts. Some of the most common uses for phytoliths in archaeology are: (1) the identification of plant taxa utilized at archaeological sites in studies of diet, farming practices, foddering, and construction materials; (2) detection of plant parts used for determining ancient activities, such as husks from grain-storage areas or plant stalks used for matting; (3) determining the seasonality of site occupations by examining the ratios of grass floral parts to stem segments; (4) the reconstruction of off-site vegetation changes in the past, from phytolith assemblages in lake-cores; and (5) identifying the earliest appearance of cultigens such as maize in South America, rice in China, and bananas in Africa. Some less traditional uses of phytoliths include: (1) evidence for both natural and human-induced fire ecology by distinguishing phytoliths from burned material versus those from unburned deposits; (2) analysis of phyto-lith properties indicating irrigation agriculture in alluvial valleys versus dry-farming in interfluvial settings; (3) identifying the first African cattle pastoralists from phytolith densities in animal pens; (4) reconstructing climatic records from carbon and oxygen isotopes found respectively within organic matter occluded inside phytoliths, and oxygen from the SiO2 itself (see Stable Isotope Analysis); and (5) direct radiocarbon dating of organic matter trapped within phytoliths (see Dating Methods, Overview; Carbon-14 Dating).
The term ‘phytolith’ comes from the Greek words for ‘plant stone’. They are mineralized particles that form within living plants and can consist of calcareous or siliceous materials. Most phytoliths are primarily inorganic and form from amorphous silicon dioxide (SiO2) which is precipitated from soluble monosilicic acid derived from the soil water. Plants commonly deposit silica within epidermal cell walls forming an exact replica of the cell, but some plants produce silica bodies in other localities as well. The uptake and precipitation of plant silica is both passive and active. In grasses and sedges, silica is deposited within plant hairs and short cells (also called ‘silica cells’) in a manner controlled by the plant physiology. Other cells can become silicified due to environmental conditions controlled by temperature, evapotrans-piration rate, soil texture, water availability, and the quantity of dissolved silica in the soil water.
Both monocotyledons (grasses, sedges, and palms) and dicotyledons (woody, herbaceous plants) produce phytoliths, but generally, phytoliths are far more abundant within the monocots. Since many genera of monocots were among the most useful plants in antiquity, the phytolith record can contribute a great deal to studies of palaeoethnobotany (see Paleoethnobotany). Monocots can be easily distinguished from dicots since the monocots are typified by a number of forms recognizable as fossilized epidermal cells which recur with regularity, whereas dicots often produce irregular shapes that do not recur repeatedly. This is a valuable distinction for environmental studies and allows the researcher to track grassland/ forest boundary shifts through time from lake cores and sediment sections in ecotonal areas. In archaeological sites, this distinction helps reconstruct the general vegetation in the area around the site as well as indicates the types of fuel used for hearth fires.
Within the monocots, phytoliths take two main forms which include the single-celled phytoliths consisting of individual disjointed cells, and the multi-celled forms (or ‘silica skeletons’) that consist of two to hundreds of adjacent cells silicified as a single body. The single-cell variety are by far most common in grasses from temperate climates, whereas the multi-celled forms are very common in areas with high evapotranspiration rates, primarily semi-arid environments. This phenomenon is enhanced under circumstances in which the monocots grow in heavy moisture-retaining soils, or under conditions of irrigation in alluvial valleys.
Phytoliths are easily recovered from archaeological deposits. A small amount of sediment (from about 2 to 30 g) is collected with a clean utensil and placed in a sealable plastic bag. In the laboratory, researchers extract the phytoliths by removing calcium carbonate residues, organic matter including micro-charcoal, and sediment material that is less than 2 pm in size (clay particles) or greater than about 500 pm (medium sand and larger), thus keeping the silt and fine-sand fractions of the sediment. This is floated in a heavy-density liquid that is calibrated to separate out the light opaline phytoliths from the quartz and heavy mineral material. The remaining phytolith residue is washed and mounted on microscope slides. These slides are usually examined under a microscope at 400 X magnification and phytoliths are counted. The resulting counts can give either relative percentages or absolute numbers per gram sediment depending upon the research methods.
The process of identification of phytoliths is different for single-celled forms versus multi-celled types. Single-celled types include forms traditionally called saddles, bilobes, crosses, bulliforms, rondels, cones, and other such terms denoting shape (Figure 1). In a very general way, some of the traditional categories of shapes used to designate single-celled phytoliths are broadly related to plant family or subfamily. For example, cones are most often associated with the sedge family (Cyperaceae). Within the grass family (Gramineae), rondels are commonly formed in pooids (festucoids), bilobes in panicoids, and saddles in the chloridoid grass subfamilies. These distinctions have allowed phytolith researchers to determine the likely environmental conditions represented by these types. Sedges are associated with moist micro-environments and standing water, pooids are C3 plants found in cool moist environments and high elevations. Chlor-idoids are C4 plants associated with warm, sunny, arid to semi-arid environments, whereas panicoids are found in warm/moist environments such as tropical regions or marshy areas in semi-arid environments. These designations are very useful environmental
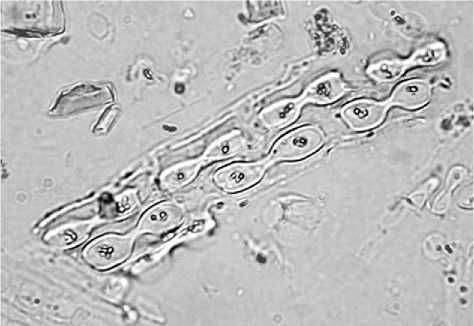
Figure 1 Bilobe-shaped phytoliths from foxtail grass (Setaria viridis). Scale = 10 pm.
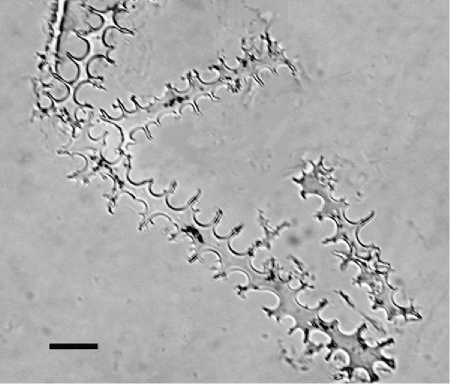
Figure 2 Dendritic phytoliths from the husk of wheat (Triticum aestivum). Scale = 10 pm.
Indicators off-site as well as within archaeological contexts. In hunter-gatherer campsites, this level of taxonomy can indicate environmental conditions at the time of occupation. In pastoralist sites, researchers can determine the micro-environments used for grazing when analyzing samples from animal enclosures or ash from dung fuels. For agricultural sites, grass subfamily might indicate the location used for agricultural fields from the subfamily of the weed grasses. More recently, there has been a concerted effort to develop an International Code for Phytolith Nomenclature in order to standardize terms used universally. This code proposes a glossary of terms relating to shape, surface texture, ornamentation, and anatomical origin.
Both single-cell and multi-cell phytoliths can also indicate the part of the plant represented in a particular archaeological sample. This is based on differences
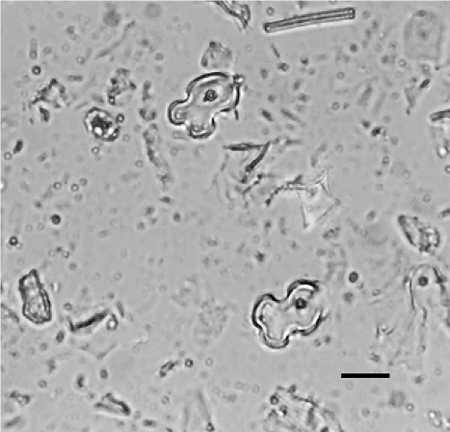
Figure 3 Variant 1 cross-shaped phytoliths from the leaves of maize (Zea mays). Scale = 15 pm. Photograph courtesy of D. Piperno.
In the shape of long-cells originating in the stems of grasses as opposed to those from the floral parts or husks of the grass. Long-cells from the stem are smooth-sided trapezoids, usually rectangular in shape. Those from the husk are dendritic trapezoids with wavy, sharply peaked cell walls (Figure 2). This distinction is very useful for determining such things as seasonality in hunter-gatherer or nomadic pastoral sites since the floral parts of grasses only appear in abundance during the flowering season, usually during the spring or early summer. In settled agricultural sites, this distinction can indicate grain-storage areas, or cereal-processing stations likely to include remains of cereal husks. Many phytoliths from smooth-sided long-cells could indicate localities at the site where large amounts of stems decayed, as in areas with matting, basketry, or roofing material.
More precise taxonomic designations, down to plant genus or even species, are also possible for single-cell phytoliths. Using multivariate and morphometric analyses of single-cell forms, phytolith analysts have been able to identify crops such as maize (crosses from leaves, short-cells from cobs) (Figure 3), rice (keystone bulliforms from leaves or double-peaked hairs from husks) (Figure 4), wheat and barley (dendritic long-cells and others), banana (short-cells from leaves), and a variety of palms.
Multi-cell phytoliths are composed of suites of adjacent cells silicified together as sheets. Since they represent an assemblage of different cell types, they can often be identified down to genus and sometimes to species. Identifications of multi-celled forms from
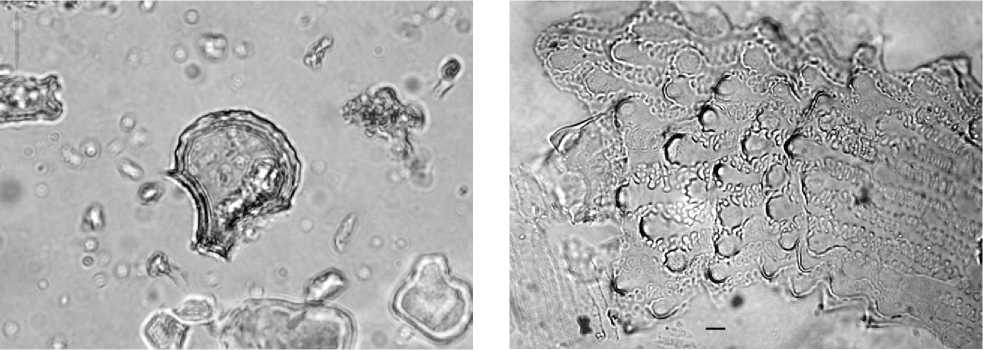
Figure 4 Keystone bulliform phytolith from rice (Oryza saliva). Photograph courtesy of E. Harvey.
Figure 7 Multi-celled phytolith from the husk of foxtail millet (Setaria italica). Scale bar equals 10 mm.
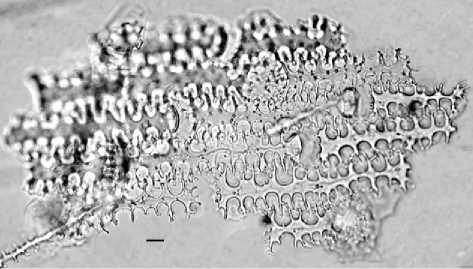
Figure 5 Diagnostic multi-cell phytolith from the husk of wheat (Triticum aestivum). Scale = 10 mm.
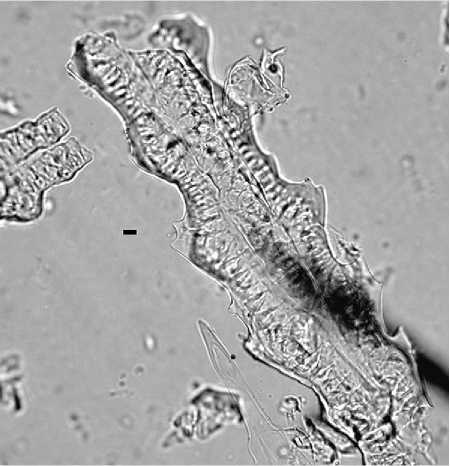
Figure 6 Multi-cell phytolith from the husk of rice (Oryza saliva). Scale = 10 mm.
Husks have been made for cereal genera including wheat (Triticum sp.) (Figure 5), barley (Hordeum sp.), rice (Oryza sp.) (Figure 6), broomcorn millet (Pani-cum miliaceum), and foxtail millet (Setaria italica) (Figure 7), and for some weed grasses including wild oat (Avena sp.), goat-faced grass (Aegilops sp.), rye grass (Secale sp.), foxtail grass (Setaria sp.), brome grass (Bromus sp.), common reed (Phragmites sp.), and others.
In addition to these more standard types of archae-obotanical studies, phytoliths have been used in palaeoclimatic reconstruction in conjunction with pollen and diatom data from pollen cores. In these studies, the distributions of phytoliths from grasses are compared with variations in tree genera from pollen to obtain a broader picture of vegetation associations from a particular region. Phytoliths from C3 versus C4 plants have also been compared with diatom data indicating lake-level changes or salinity.
When analyzed in conjunction with macrobotanical remains, phytoliths contribute additional dimensions to an overall picture of palaeoethnobotany by allowing researchers to access the record of plant shoots in addition to the record for seeds and wood charcoal. Since unlike macrobotanical materials phytoliths do not need to be charred to be preserved in the archaeological record, they contribute archaeobotanical information in unburned archaeological contexts. Finally, in many archaeological settings with poor macrobotanical records, phytoliths are the only indicators of plant use.
See also: Carbon-14 Dating; Coprolite Analysis; Dating MeThods, Overview; Macroremains Analysis; Paleoeth-nobotany; Pollen Analysis; Stable Isotope Analysis.